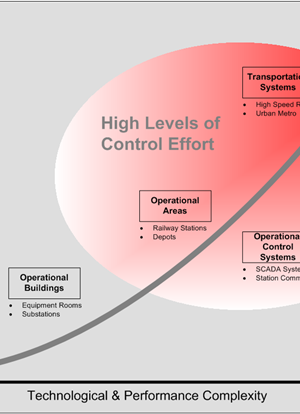
Integration, Testing and Commissioning at Crossrail
Document
type: Technical Paper
Author:
Siv Bhamra, Marios Georgaras, ICE Publishing
Publication
Date: 09/07/2018
-
Abstract
Crossrail’s Central Operating Section is characterised by high performance requirements of a metro system with high specified train service throughput. This is realised via multiple interdependent electronic systems which control the movement of trains together with station systems, traction and auxiliary power supplies and wayside equipment. These need to be integrated with the stations and other infrastructure assets to collectively provide for a safe, operable and maintainable railway. The Central Section and Network Rail interface works at Westbourne Park and Pudding Mill Lane need to be carefully planned and managed.
The paper discusses how the delivery risks can be identified and mitigated via a set of well defined commissioning strategies and the use of various management tools, processes and test facilities. Comprehensive planning is utilised to maximize the extent of trials and testing away from the operational railway.
Additionally, strategies are set by organizing and managing the use of test tracks. The necessary equipment to be installed and the types of tests to be performed are defined. Alignment of the design development of the different systems with the appropriate tests in phases is achieved to support the de-risking of the back-end commissioning of the programme.
Also, the use of a selected part of the railway to begin early testing and reliability accumulation to enable a smooth introduction of new rolling stock and achieve early systems integration experience is introduced. Its development requires attentive planning and rigorous monitoring of progress as well as early risk identification and mitigation.
Supporting the aforementioned activities and as a further de-risking tool, an integration facility (simulator) is initiated. This facility allows for intensive ‘off-site’ testing of planned timetables, validation of degraded and emergency operational scenarios, as well as supporting training and development of operational and maintenance staff.
-
Read the full document
Introduction
Technological advancements have shaped the development of mass transportation networks over more than a century. Railways have played an important role in safely and efficiently transporting hundreds of millions of people and freight all over the world since the invention of the first steam operated locomotive in the UK in the early 19th century. New technologies have changed and improved rail journeys steadily over the past two centuries.
The global application of railways and evolution into various forms to suit operational requirements demonstrates their current and future economic benefit. Today’s railways serve mainline routes between large metropolitan cities, dedicated urban light transit applications, freight networks as well as dedicated high speed lines which connect major cities across distant areas.
New technologies are being introduced yearly to respond to growing demand for safer, more efficient, operationally flexible and higher performing railways. The added commercial pressures to shrink timescales for designing and implementing new major rail projects in order to secure early revenue income is resulting in a challenging delivery environment. [1]
One of the most distinct examples of the above is Crossrail. The programme is being implemented in one of the busiest cities in the world with numerous requirements from various stakeholders. Apart from the performance and operational needs for reliability and maintainability, there are pressures for minimum disruption to existing live railways, minimum nuisance to the public and the city’s everyday life and operation, and of course the limitations derived by budget and time constraints.
In addition to these, the complexity of the railway itself, when viewed from a systems perspective, supplements to the existing pressures. Crossrail is a metro-style railway in its Central Operating Section, which then transitions to two surface lines of existing railways. The interfaces to Network Rail’s systems increase the overall delivery risk and thus need to be managed in a structured way. Among other project management processes which are being implemented (Engineering management, Requirements management etc.), Integration and Testing and Commissioning become of paramount importance, since these are typically the latest stages of the programme delivery.
Background
Rail Technology and Systems Integration
In order to satisfy the business drivers, new rail projects are increasingly being specified in a manner leading to the emergence of highly-integrated railway systems. In particular, over the course of the past 25 years the concept of ‘total rail systems integration’ has emerged. This thinking leads to the definition in Figure 1.
Figure 1. Rail Systems Integration defined
Modern rail technologies and systems are now becoming highly integrated. In revenue service these integrated systems must work effectively to support safe and efficient operation of trains, stations and other service infrastructure. They also provide timely and accurate information to users, assist operators during any service disruptions as well as provide ancillary services such as operational performance information.
Modern railway control systems are increasingly integrated to provide the following essential operational functions, and of course Crossrail is no exception:
- Signalling and train control – ensures safe separation between trains and provides for efficient movement of trains
- Traction power control – monitors and controls traction power and high-voltage inputs to the railway and may even regulate the receptivity for regenerated power
- Passenger information and public address – provides visual and audio information on train arrivals, departures and other details of services on-board
- Telephone and radio communications – allow to transmit the information necessary to control railway operations
- Ventilation and environmental control – provide monitoring and control of ventilation, heating and air conditioning, lighting, drainage and fire fighting systems in railway tunnels, stations, control and equipment rooms
- Closed-circuit television (CCTV) and passenger assistance – provides visual monitoring of railway premises and information to passengers, particularly for assistance and support
- Fire and security – monitor fire and security hazards
- Station management systems – provide station monitoring and control, including lifts, escalators and equipment rooms
- Automatic revenue collection – provides secure revenue collection and associated records
- Remote condition monitoring – provides data on the performance of major infrastructure, including static assets such as bridges and embankments
The above systems can be brought together to form an overall Integrated Control System, which is a major challenge at Crossrail and obviously at any other programme of this scale.
Managing Technological Complexity
Balancing System Integration Complexity with Control Effort
The level of management control effort has to be balanced against the understanding of system integration risks to project outcomes. If a project has key systems which are not effectively integrated then there will be implications of cost increases, delayed provision of the railway service to full functionality as well as concerns over future safety, reliability and maintainability. There could also be longer term ramifications of reputation damage and loss of political and other stakeholder confidence.
As the level of systems integration complexity increases, it is necessary to dedicate the appropriate level of highly competent leadership and supporting technical expertise. For comparatively less complicated civil engineering structures and buildings the level of integration complexity and associated risk is relatively contained. However, as advanced technologies are brought together to form highly integrated systems, as is the case at Crossrail, the need for dedicated effort towards integration becomes essential. Figure 2 shows the relationship between the technological and performance complexity and the corresponding systems integration control effort to manage the risk. Beyond a certain threshold it becomes necessary to appoint a dedicated and highly experienced team to manage the systems integration effort. [1]
Figure 2. Relationship between Integration Complexity and Control Effort
Integration
The left-hand portion of the “V” diagram, shown in Figure 3, depicts the flow of concepts and requirements being captured, derived, expanded and allocated to design elements in an increasing level of definition and detail, culminating in the implementation and fabrication of all the subsystems and elements. The right-hand side of the “V” diagram describes the test, integration and validation of these sub-systems into a working railway.
The mapping across from each side of the V points relates to the fact that a successful testing and commissioning phase is dependent equally on the quality of the design phase as to the processes and their adequate implementation in managing test and integration.
The delivery team relies on the expertise of its systems integration engineers and the empowerment of stakeholders to formulate an effective test, integration, and commissioning strategy which fully encompasses the railway system and its main interfaces, such as existing infrastructure, existing systems and operational and maintenance procedures and facilities.
This strategy must address the way in which the various elements will be integrated and contains a basic testing and commissioning structure to be used to deliver a railway fit for robust operational performance.
System interface definition may be used to define the boundaries between sub-divisions of an elementary system and determine the commissioning lots in order to ensure that testing is exhaustive. It also serves to prepare the Integrated Logic Diagram, describing the order and sequence of testing subsystems and systems and their tie-ins, logically sequenced to meet the overall programme key dates.
Figure 3. Managing Integration through Project ‘V’ Life-Cycle Stages
At Crossrail, in order to overcome some of the difficulties of on-site integration and mitigate the associated risk, the strategy maximises factory and off-site integration testing to prove functionality and interfaces before equipment is delivered to site. Equipment is delivered to site tested and configured. Thus, extensive use must be made of simulators and other test facilities including utilising a temporary control module. The balance of options available between actual and modelled/simulated proving is shown in Figure 4.
Figure 4. System Development and Proving
One of the tools that can be used to manage the integration process is a failure reporting, analysis and corrective action system, often implemented using a database, accessible from remote sites, providing a facility for reporting and classifying failures during the integration phase, and for planning corrective actions in response to those failures. It can also be used to provide metrics and performance indicators.
Testing
The purpose of Testing is to expose subsystems and integrated systems to a range of investigations and trials to reveal their performance under controlled conditions against expected behaviour.
Testing is closely aligned to the integration effort, and follows a structure to demonstrate progressive compliance leading to commissioning. The phases at Crossrail comprise:
Factory Acceptance Tests validate each sub-system once completed by the manufacturer against requirements, design intent, and standards. Typically the sub-system under test is within an environment of simulated interfaces, drivers, and recording devices.
Static Tests are undertaken when all sub-systems are installed and connected in order to verify they work in accordance with the design and function as demonstrated previously in the factory, and on an integrated basis. Interfaces between the different systems involved are tested by simulation because external systems are not typically connected at this stage.
Static Integration Tests are undertaken when the interfaces between all systems, including the interfaces with the systems of third-parties such as the external railway networks are fully connected. The integration testing regime will follow a defined process that would allow for the progressive proving of technological or performance functionality over an increasing level of geographical area. Defined railway baselines will act as control points to confirm the planned integration functionality at each test stage has been reached. This concept of progressive integration proving is shown in Figure 5.
Figure 5. Progressive Integration Proving
In Dynamic Integration Tests, the process starts with a test train running under controlled conditions. The purpose is to verify that the design and installation of the railway systems comply with their requirements, and that all systems interfaces function and are integrated so that the overall system operates properly and safely to the appropriate standard and in accordance with the requirements.
Trial Runs will involve appropriate integrated testing with trains to demonstrate that the operating railway system is capable of reliably meeting the capacity and other requirements of the programme. Tests will include trials to demonstrate minimum headways, journey times and capacity, plus the ability of the system to operate in degraded modes. During this phase, the railway will be operated under defined rules.
Trial Operations cater for full running of the railway in shadow passenger operations. This phase enables the operators and maintainers to conduct final preparations before opening the railway to revenue service. This phase also gives opportunity to operators and emergency services to test their responses to scenarios resulting in major service disruption or incidents. The output of these scenarios can be used to update the operations and maintenance response procedures.
Commissioning
Through strategic planning and subsequent supervision, there is the opportunity at the commissioning stage to significantly de-risk the project, notably through phased opening of Crossrail and sequenced interface commissioning with existing operational assets and systems.
Testing and Commissioning Strategies
The team uses this structured framework to deploy specific and targeted testing and commissioning strategies in order to de-risk the delivery of Crossrail into passenger service. A Test track, the integration facility and an advanced proving section of the railway form the basis on which these strategies will be deployed.
Test Track
The rolling stock supplier typically has access to one or more dedicated test tracks for early systems integration and factory acceptance testing, either as part of its manufacturing facilities or in close proximity. As the research, development and design of the new train progress, the team gains increased visibility of the systems which will need to be tested on the test track and their functionalities. The intent is to test and prove the basic systems (mechanical, electrical, on-board signalling) of the new trains in a controlled environment and begin preparation to secure the necessary regulatory and operator approvals before introducing the new rolling stock to the newly constructed, upgraded or existing infrastructure. This will be achieved by recreating the behavior of the different systems and testing the integration of the rolling stock, signalling and control and infrastructure elements of Crossrail.
Ideally, the development and use of a test track will be embedded in the suppliers’ contracts from the outset. This enables the technology providers to align their systems development and testing programmes and simplify or even eliminate any additional commercial arrangements required. Most importantly, it makes engagement and buy-in for the implementation of this strategy straightforward.
The team facilitates the coordination between the technology and systems suppliers and the exchange of information. It would be prudent to initiate this process relatively early during the design of all the systems (rolling stock, signalling, communications) in order for all parties to get familiarized with the systems and start forming collaborative, professional working relationships with each other. Figure 6 shows the application of testing requirements in the full V-life cycle of major railway projects and how it is being implemented at Crossrail.
Figure 6. Testing Requirements in Project V-life Cycle
The elements which need to be taken into consideration when forming the strategy and programme of testing at the test track are the systems development maturity level, availability of test track and access arrangements, software releases and relevant functionalities, driver training and maintenance facilities.
It is important that the test track tests are timed in a way that useful results can be obtained to support further systems development and integration and software fault-finding and development. Further, miles accumulation and factory acceptance tests will support the process of gaining the appropriate certificates and approvals for the next phases of testing.
The system suppliers meet regularly at technical and management workshops with the active engagement of the delivery team in order to finalise the scope of testing and the test track’s design. Decisions need to be taken for the length of the test track and the equipment to be installed (signalling and control, communications, radio etc.) based on the estimated progress of the systems development and the testing programme. This process needs to include both design and testing and commissioning professionals to make sure that the design and tests will reflect and be representative of the real equipment and software which will be used in passenger service. The output of these workshops will be the test track specification detailing the design which is appropriate and feasible for implementation.
The specification contains all the necessary information to build the test track. It outlines the purpose, scope, limits and boundaries of the track, assumptions made, external dependencies (planning permissions), third parties and stakeholders’ input and required engagement.
Once the specification is finalised and all parties’ input and feedback have been taken into consideration, the next phases commence. The suppliers will procure the necessary equipment to fit out the test track and plan in detail the tests which will be performed. These will typically be the different driving modes and the transitions between them (manual, automatic, protected manual, auto reverse etc.). The dynamic tests will progress further by testing in increasingly higher speeds and testing the transitions between different signalling systems.
Integration Facility
Typically, factory and test track testing by suppliers will test real systems to simulated interfaces and in particular the interfaces which are of bigger interest to the systems providers. In complex railway projects and Crossrail, there is still much risk left for the later stages of testing and commissioning to complete the interface testing and integrate the critical systems. In an effort to de-risk the back end of the programme, the Crossrail Integration Facility was deployed.
The integration facility brings together systems from the main suppliers (rolling stock, signalling and control, platform screen doors) into a controlled environment. The aim is to test the functionality and operation of the critical signalling and train control systems. It comprises a mix of real production hardware and software, equipment simulators, a train cab mock-up, a traffic and train movement simulator and a user environment.
The key benefits of the integration facility can be:
- It enables the critical railway systems interfaces to be stressed in terms of loading the system with large numbers of train movements, injecting failures and debugging the system far in advance of running large number of trains on the live railway
- It enables certain tests to be performed, e.g. failure testing, which cannot easily be performed on the live railway
- It is easier to debug and diagnose faults
- It enables early integration testing of real equipment in a controlled environment
- It allows system baselines configurations to be proven and managed prior to release to the field
- It allows for failures during dynamic testing to be reproduced, diagnosed and re-tested quickly and without having to book testing access
- It provides early visibility and familiarization for the operators and maintainers of the operation of the systems across the transitions
- It provides early visibility and familiarization of the equipment for the maintainers
Apart from the immediate benefits of the integration facility, there are more opportunities identified in its use. Although it is not a training simulator, it could be used to mitigate delays of late delivery of training simulators. Also, beyond the life of the construction of Crossrail, it can be used to test planned upgrades of software and data to maintain a reliable and robust operational system configuration.
The functions of the integration facility were developed within the project team by means of an analysis of technical interfaces, with interfaces defined as novel, complex or mission critical considered for inclusion within the facility’s functions. Mechanical interfaces were excluded from the scope and lesser priority was given to interfaces that remain within the scope of individual suppliers.
For the user interface, the principles followed are:
- The facility provides for simulation to be undertaken via test scripts so virtual trains can be forced to run to a particular script and not to the timetable
- It will be possible to force one or multiple faults in the simulation. These faults will be any which impact the signalling and control system
It was critical to time the development of the integration facility in a way which will maximize its use and subsequent benefits. If it was done too early, the suppliers’ systems would not be mature enough to be added to the facility. If it was done too late, the use of the test track and the dynamic testing phase itself would diminish the value of the integration facility in de-risking the opening of the railway.
The Crossrail project team with the support of the systems specialists wrote the specification of the integration facility. This details the purpose and scope of the lab and outlines the high level requirements for its operation. It also contains a basic system architecture and the list of deliverables for the design phase.
It was then possible to publicize the specification and receive quotations for the design and build of the system (as the facility was not already part of the suppliers’ contracts). Because of the complexity of the real railway and the suppliers involved (scope-systems, responsibilities, timescales) the project team had to instruct a specific technology provider to proceed with the implementation of the facility, without receiving bids from others.
There were specific benefits in doing so and other risks by competitively bidding. For example, the signalling contractor was instructed to design and build the facility, so immediately all the signalling intellectual property issues were solved. Also, the signalling project team already has large knowledge of the system and can engage and progress swiftly to the design and build. In contrast, if an external to the project supplier was appointed (obviously a competitor to the current suppliers as well) it would be very time and money consuming to gain the same level of knowledge of the systems, establish the right working relationships and deliver in a tight and pressing time schedule.
Another important aspect of the integration facility which needs to be taken into consideration with regards to the timing of its design, build and subsequent deployment is the programme of software and data releases for the main railway project.
By definition and specification and in order for the facility to serve its purpose and be as representative as possible of the real system, it needed to contain the same hardware and software configuration as the railway. The challenge is that the integration facility would need to be delivered in advance of the project completion but at the same time offer the opportunity to the test engineers to use a system which is very close to the final product.
Thus, the software and data development releases from the different suppliers needed to be known and factored in the facility’s design and build programme. In this way, it is possible to incorporate meaningful configurations, allow the facility’s builder to integrate the different systems and enable the users to perform useful tests. In later stages of the project, new releases will be used as the suppliers’ systems become more mature and testing progresses.
Advanced Proving Section
Experience from large railway schemes has shown that the testing and commissioning phase is usually compressed at the back-end of the programme. There are many reasons for this; delays in design and construction, changes, integration problems etc. In order to mitigate the risk of further delays in the testing and commissioning phase which could affect the date of opening for passenger service, the railway advanced proving section is introduced. For Crossrail, this is formed by the section from Abbey Wood to Canary Wharf Stations.
This is effectively a section of the whole railway which will be completed ahead of the other parts and has certain characteristics which will allow dynamic testing to take place:
- It provides access for the test trains to enter the section and a temporary or permanent maintenance facility
- Equipment rooms and essential services at the respective stations, shafts and portals are complete
- It provides stabling facilities
- The high voltage traction and non-traction power is available
- The fibre and data network are available
- Installation of systems (signalling, communications, overhead line equipment etc.) is complete
The critical dependency for the commencement of dynamic testing is the energisation of the overhead line equipment. This process requires the coordination of works and systems across multiple contractors to prove the safe operation of the traction power and also requires consultation with a number of key external stakeholders.
The key steps are:
- Issue notification of energisation to local authorities, external railway networks and external utility networks
- Perform a hazard analysis to identify the potential risks and ways of mitigating them
- Perform insulation and continuity tests on dead catenary before energisation
- Take possessions on adjacent railway networks with exclusion zones during the energisation, section proving and short circuit testing if the hazard analysis requires it
- Arrange for observation teams in signalling rooms, control centre etc. and anywhere considered sensitive as a result of the hazard analysis
- Take EMC and touch potential measurements during both energisation/section proving and short-circuit tests with quick analysis
It is important that there is a very clear and communicated responsibilities matrix for this phase. There are many activities which need to happen in specific order and which involve many different parties. The main ones are:
- Test management and coordination
- Test logistics and planning
- Rule book issue
- Train servicing and maintenance
- Train drivers training
- Preparation for and timely submission and acceptance of certificates and regulatory approvals
- Third party agreements and notifications
The testing and commissioning team with the endorsement of Crossrail’s leadership, organizes a series of planning workshops with the systems contractors. In these meetings, there are discussions over the commissioning logic and systems architectures. The aim is for the contractors to create an integrated testing and commissioning plan taking into consideration all the parameters, restrictions, interfaces, dependencies and assumptions which apply.
The dates which will emerge from the integrated testing and commissioning programme need to be fed back to the contractors’ main programmes. It is anticipated that this process will uncover discrepancies between the two programmes and probably installation dates and sequences will need to be changed. It is important to align the contractors to comply with the integrated programme in order to achieve the benefits of the advanced proving section testing.
Fig. 7 shows how the use of early test initiatives at Crossrail, such as the Test Track, the Integration Facility and the Advanced Proving Section can help bring forward development and deployment of de-risking solutions to early stages of the project. This in turn will reduce risk exposure to later stages of testing and commissioning the systems.
Figure 7. Risk Mitigation Strategy through Early Testing
Conclusion
In complex programmes such as Crossrail, a multitude of interdependent systems need to be integrated and tested in order to provide for a reliable service of the railway under development. In addition to the technical requirements and derived challenges, the presence and influence of multiple stakeholders need to be recognized and addressed. Therefore, it is prudent to begin early identification of risks and implement associated control measures to manage the delivery of the railway in a structured way. Integration, Testing and Commissioning need to be actively planned and managed from the outset of the programme. The goal is to support the delivery of the programme by de-risking its final stages through a set of different but linked strategies which require attentive planning and coordination by highly competent and experienced commissioning engineers. At Crossrail, the Test Track, the Integration Facility and the Advanced Proving Section are the initiatives deployed to underpin the management effort of completing such a complicated programme within its time and budget constraints.
References
[1] Bhamra, S., Georgaras, M. (2014). Systems Expansion whilst Minimising disruption to existing operations. AfricaRail Conference 2014
-
Authors
Siv Bhamra - Bechtel, Crossrail Ltd
Siv Bhamra is a senior executive with 30 years of experience in the project management and engineering of major rail projects. He has worked on the full spectrum of rail projects from light rail, urban metros to high-speed lines, engaging in activities ranging from conducting feasibility studies to implementing full schemes. Siv has delivered rail projects in Europe and the Far East and has performed studies for rail operators in the USA, Middle East and South Asia.
Siv has worked on the Jubilee Line Extension Project in London. Before that, he had worked in senior roles with London Underground and a number of railway companies. Siv also served 2 years as principal transportation advisor to the European Bank for Reconstruction and Development. Currently Siv is Rail Systems and Commissioning Director on the Crossrail Project.