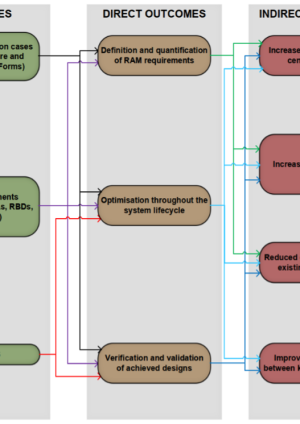
RAM in an Interconnected World
Document
type: Technical Paper
Author:
Paul Muchatuta BEng MSC MIET, Tomas Cuesta Brooke-Turner MEng MIET
Publication
Date: 02/12/2021
-
Abstract
Sustainability and Reliability, Availability and Maintainability (RAM) are two different means to the same end: conservation of resources. In the case of sustainability, these resources typically relate to environmental, economic, and social outcomes, whereas in RAM it relates to the design optimisation of systems. There are three levels of impact that should be considered to fully assess successful RAM contribution – Direct Outcomes (interaction within systems), Indirect Outcomes (resulting from the project), and Induced Outcomes (actions of projects at a more holistic level).
The implementation of RAM on Crossrail Systemwide contracts provides examples of how inherent RAM Systems Thinking was utilised in the optimisation of a major infrastructure project throughout its system lifecycle. It involved the definition and quantification of RAM requirements, then the verification and validation of achieved designs against those performance objectives, using RAM analytical techniques, modelling, and empirical site data from DRACAS.
On the Indirect Outcome level, RAM has delivered a high performance resilient railway that supports the TfL sustainability framework targets of Economic Progress and Climate Change. Finally, on an Induced Outcome level, the success of RAM drives development of social outcomes in London via the achievement of UN Sustainability Development Goals 8, 9, and 11.
-
Read the full document
Executive summary
The aim of this paper is to describe the Systems Thinking principles of Reliability, Availability and Maintainability (RAM) engineering carried out throughout the lifecycle of the Crossrail project, and the key contributions of these processes to the successful outcomes resulting from the delivery of the railway. The main focus is the RAM activities applied within the scope of the Central Operating Section (COS) of the Crossrail project, and its constituent tunnel and rail systems. Additionally, the paper identifies the direct links between the RAM principles and theory, the technical activities and deliverables, and the resulting impacts and outcomes of the project.
This technical paper highlights the importance of the application of RAM engineering to the successful delivery of a complex multidisciplinary project. Furthermore, its contributions are not exclusively limited to the Direct Outcomes of a project but extend outwards in the form of Indirect and Induced Outcomes, the impact of which have Social, Economic and Environmental considerations.
It has therefore been concluded that a comprehensive adoption of Systems Thinking of application of RAM engineering has proven to be extremely beneficial for the purposes of successful project delivery. Furthermore, it can be confidently asserted that the importance and added value of these approaches will undoubtedly grow in future projects in an increasingly interconnected world.
1 Introduction
The Crossrail project is a capacity enhancement rail project tasked with linking the west of London, from Reading and Heathrow, to the east and south east, through to Shenfield and Abbey Wood. The completed project, as seen in Figure 1, delivers the Elizabeth line, the first completely new underground line through central London in over 30 years. For many years Crossrail has been one of the biggest railway infrastructure projects in the world, in part due to this undertaking requiring the laying of 118km of rail track, the complexity of interweaving with national rail services on Network Rail infrastructure, as well as the consideration of passenger flows in the new dedicated sub-surface infrastructure throughout central London at connections with London Underground (LU) and Docklands Light Railway (DLR).
Figure 1. Crossrail Project and Central Operating Section
The Alstom TSO Costain Joint Venture (ATC) C610 Systemwide Main Works contract concerned the design; procurement of plant, materials, and equipment; installation, commissioning and testing of the high capacity, metro-style Central Operating Section (COS). Mott MacDonald (MMD) has supported the ATC contract via the MMD scope of work, consisting of developing the Tunnel Ventilation System (TVS) and Tunnel Mechanical and Electrical (M&E) Systems. Furthermore, the MMD Reliability, Availability and Maintainability (RAM) team has had the unique opportunity to support ATC in conducting RAM Engineering on the entire C610 scope. This included Track and Overhead Line Equipment (OHLE) systems, as well as the C644 Traction Power Infrastructure; C650 Non-Traction High Voltage (HV) Power; C695 Plumstead Maintenance Facility; and C696 Plumstead Stabling Sidings contracts.
The purpose of this technical paper is to present the methodology of the integrated RAM engineering undertaken throughout the project lifecycle and arguments for the hypothesis of beneficial outcomes that have arisen as a result of this novel approach. Using the Theory of Change outline, this paper will examine the outcomes that have arisen at three main levels:
- Direct: Beneficial outcomes of RAM engineering involvement on the design of the system and subsystems.
- Indirect: How RAM engineering involvement has contributed to the construction of a highly reliable and resilient railway, and the resulting beneficial outcomes.
- Induced: How RAM engineering involvement has contributed to the development of Social, Economic, and Environmental outcomes external to the immediate scope of the project.
To reflect the scope of this paper, the content has been structured to capture the following:
- RAM Procedure/Method – This section introduces basic RAM theory within the broader scope of Systems Thinking, and outlines details of the implemented RAM programme.
- Results, Discussion, and Analysis – This section presents the main findings and comments on their significance in impacting sustainability.
- Lessons Learned and Recommendations – This section presents a number of lessons that have been learned and how they can be successfully applied in the future.
- Conclusion – A summary of the hypothesis, findings, and interpretation of the results.
The procedure and a generic example of the RAM programme that was implemented is provided in Section 2.
2 RAM Procedure/Method
RAM (Reliability, Availability and Maintainability) engineering is a key part of a broad and holistic approach to the integration of subsystems known as Systems Thinking. Systems Thinking offers a methodology that is based on the belief that the component parts of a system will act differently when isolated from the system’s environment or other parts of the system. As a result, consideration of the emergent properties and behaviours of complex system are at the core of a Systems Thinking approach. This offers a contrast to traditional analysis which prioritises the breaking-down of complex systems into separate elements for isolated study and consideration.
2.1 RAM Theory Fundamentals
Reliability, Availability, and Maintainability are system design attributes that have significant impacts on the sustainability and total Life Cycle Costs (LCC) of a developed system. A system’s RAM attributes contribute to the optimisation of the design, to achieve the balance between the system’s performance and the use of resources. The standard definition of the distinct areas that are covered under the scope of RAM engineering are:
- Reliability: The probability of failures over a defined time interval. The key metric for reliability is the Mean Time Between Failures (MTBF).
- Availability: The percentage of time a system is considered ready to use when tasked. The key metrics for availability are the MTBF and the Mean Time To Repair (MTTR).
- Maintainability: The measure of the ease and speed with which a system or equipment can be restored to operational status following a failure. The key metric for maintainability is the MTTR, a subset of which is the Mean Active Repair Time (MART).
In order to maximise the added value of RAM engineering, it should be applied throughout the project lifecycle. A simplified graphical representation of a systems development cycle can be seen in the in Figure 2. For the purposes of simplicity in outlining the standard application of RAM engineering as outlined in BS EN 50126[1], the lifecycle of a project can be divided into:
- Understanding the problem space: Development of RAM strategy (captured in a RAM Management Plan), which aims to achieve the identified overall RAM requirements of the system.
- Detailed design and construction: Using the system’s RAM requirements, individual targets are derived and apportioned for specific subsystems and equipment. As the level of maturity of the design increases, so does the accuracy of RAM performance predictions and estimates, making RAM engineering a key contributor to the technical design process.
- Integration, testing and commissioning: Once the construction and installation phases have been completed, the integration of the subsystems and designs can be performed, resulting in the completed system entering operational service and performing its intended function.
- Assessing system value: Throughout these phases, assessment of the RAM characteristics of the completed system, verification and validation exercises must be carried out to ensure that all RAM requirements have been met and that the delivered system is highly resilient and reliable.
Figure 2. Simplified systems development lifecycle diagram
2.2 Application of RAM Engineering in Crossrail
RAM engineering has played a crucial role in the application of Systems Thinking throughout the lifecycle of the Crossrail project. The process that was adopted is aligned with British and European standard BS EN 50126[1]. As a result, RAM engineering has provided valuable technical inputs during the establishment of initial RAM requirements and targets for Cross London Rail Links (the precursor organisation to Crossrail Limited) in 2006, through to the development of the Crossrail Works Information documents during the detailed design stages, to the testing and commissioning of the multitude of railway systems.
2.2.1 Development of RAM Plan and targets
During the early stages of the project, a key emphasis was placed on essential activities surrounding the development of the RAM Plan, the RAM performance target allocation (both quantitative and qualitative), and the RAM assessment activities for Cross London Rail Links. The importance of the foundations of the RAM process that were developed throughout these early stages were of critical importance, since they provide the basis for future refinement through to the project completion stages. Additionally, a comprehensive understanding of the planned RAM activities and processes by all project disciplines was necessary, in order to maximise the added value of RAM engineering on the project outcomes. Challenges were in fact encountered in this domain due to unfamiliarity with the concept of Systems Thinking and their importance to project success and the more tangible milestones, however they were surmounted via proceeding with a collaborative, influencing approach as opposed to the more prescriptive, directing approach often found in main stays of engineering projects.
2.2.2 Use of RAM to inform System Design
RAM activities during these stages focused on the planning and delivery of the RAM engineering and assurance activities for the MMD reference design packages, including the main C124 Rail Systems contract. This included the apportionment of subsystem-specific RAM requirements and contributed to the detailed design of the elementary subsystems.
A collaborative and iterative process was followed, the basis of which involved the gathering of designs and architectures for the elementary systems. These were subjected to continuous analysis for estimated RAM performance based on the proposed components, equipment and architecture. Additionally, while evaluating all proposed designs, the overall Life Cycle Cost (LCC) was also included under the RAM scope.
The RAM activities focused on the study of the proposed design performance and provided technical input to aid refinements to the design as its level of maturity increased. This was done by analysing the effect of potential failures of all subsystem assets, corrective and preventive maintenance times and redundancies built into the proposed design.
Throughout this process, a number of analytical and computational tools were used to assist in the RAM performance modelling of individual subsystems. These included:
- Qualitative RAM performance analysis processes to identify all relevant failure modes, mechanisms and causes, and determine any resulting maintenance and testing strategies and considerations:
- Functional Block Diagrams: System model which includes its constituent components, its boundaries and interfaces, and its functions under various system operating modes.
- Failure Mode, Effects and Criticality Analysis (FMECA) to identify the potential failure modes within the systems and to determine their causes and effects.
- Reliability Critical Items List (RCIL) to identify items with relatively high failure rates, possible obsolescence, short life expectancies, difficult to maintain, or had novel features, any of which has the potential to reduce system availability.
- Quantitative RAM performance modelling and prediction techniques to produce performance estimates of the proposed system design:
- Fault Tree Analysis (FTA) tools: Approach that aimed to quantify the reliability and availability of a complex system through the use of Boolean logic gates and the study of individual component failure rates.
- Reliability Block Diagram (RBD) tools: Diagrammatic analysis method for demonstrating component reliability interaction within a system and the effects system design and architecture have on performance and redundancy.
Figure 3. Basic equivalent FTA and RBD examples
- Iterative calculation techniques, like Reliability Growth Management, defined as the process of planning, measuring, and monitoring the increase, or otherwise, in reliability achieved through the application of the DRACAS process, further expanded upon in Section 2.2.4.1. Carried out by using the Duane method[2], plotting the failure rate data against cumulative operating time on a log-log graph.
In addition to these analytical methods to study the RAM performance of the elementary sub-systems, the overall achievement of the RAM requirements was dependent on the performance of the interfaces between the systems under the C124 scope and those under other contracts. As a result, a key part of the RAM engineering activities revolved around the effective interface management with external systems, and included the targeted study of RAM performance at key interfacing points.
2.2.3 Construction and Installation
The application of Systems Thinking throughout the final stages of the project lifecycle focused on the delivery, management and technical support surrounding the RAM processes and deliverables. These included providing RAM performance engineering and assurance support for the construction, installation, testing and commissioning phases for the COS railway systems, and the collection and study of operational data required for the processes of verification and validation.
2.2.4 Assessing System Value
Once each of the elementary subsystems had been commissioned, the operational data acquired throughout the installation and testing phases was used to verify the accuracy of the RAM performance predictions developed throughout the project lifecycle. This provided assurance that the RAM targets had been met. A key tool in the acquisition and analysis of operational RAM data was the Data Reporting and Corrective Action System (DRACAS).
2.2.4.1 DRACAS Database
DRACAS is a closed loop database tailored to the reporting and analysis of incidents, defects, faults and failures, as well as the corrective actions that have been implemented as a result of these occurrences. The system is intended to provide validation and increase the confidence in the accuracy of the claimed theoretical RAM performance analysis.
DRACAS was adopted as part of the Crossrail project in the final stages of installation and during testing and commissioning, in line with the bespoke guidance produced for this process[3]. A simplified diagram of the DRACAS procedure that was adopted throughout these project stages of the pertinent systemwide contracts can be seen in Figure 4.
Figure 4. Simplified diagram of the DRACAS process
An additional purpose of the DRACAS database was to provide validation and supporting evidence that the RAM characteristics of the elementary systems met the established RAM requirements. This was done by collecting and analysing relevant fault and defect data throughout the testing and commissioning stages of the project. As a result, this operational data was used to verify the accuracy of the RAM performance modelling and analysis carried out during the design stages of the project and allowed for any required changes regarding MART and MTBF to be made to both the RAM Data Forms and the software models.
All the RAM requirements, modelling, analysis, and theoretical and operational data was compiled, examined, and presented in RAM documentation for each elementary subsystem, which was issued at works completion. This provided evidence of compliance derived from the RAM engineering activities, and supported the contention that each defined elementary subsystem satisfied the RAM requirements and provided an audit trail of the engineering considerations from requirements through to evidence of compliance.
3 Results, Discussion and Analysis
This section presents the main findings and synthesis on their significance in impacting sustainability using the Theory of Change methodology[4]. A Theory of Change inspired diagram has been included in Figure 5 to graphically present the results using a Systems Thinking approach. It should be noted that the interdependencies between the RAM workstream and the overarching Induced Outcomes is highly complex beyond the depth of granularity possible to cover in this paper alone. However, an effort has been made to explore the relationship between them.
Figure 5. Theory of Change Inspired Diagram
3.1 Direct Outcomes
The Direct Outcomes reflect the beneficial consequences of RAM involvement in the design of the systems, using applied systems engineering principles to inform and affect the design.
The first step in this process, as is clearly presented in the Systems Engineering V lifecycle shown in Figure 6, is understanding the problem space. Understanding the problem space was achieved by utilising the technical RAM requirements as presented by the Crossrail COS Works Information Requirements and Crossrail Systems Engineering RAM Plan, as was applicable to each system. The RAM Plan was used as a vehicle to agree requirements and interpretation of requirements in relation to specific systems that they were apportioned to, both in terms of definition and the specifics of how they were to be quantified. This process required an ongoing trade-off analysis between the desired effect of the system, budget constraints, and technical feasibility. The results of this were circulated to relevant stakeholders to ensure that they were able to input into the process, thereby utilising collaboration to minimise the risk of not achieving the desired system purpose.
Figure 6. Systems Engineering V Lifecycle model[5]
This progressed into understanding the solution space, with the investigation of potential solutions being explored. In terms of RAM specific activities, this included the apportionment of RAM requirements to suppliers as part of the tender package. In order to be compliant, suppliers were required to provide equipment which was in accordance with the RAM requirements. This compliance was used, alongside numerous other design factors, to determine a preferred solution. An example of how RAM engineering informed this decision would be the usage of RBDs and FTAs to assess system on-demand availability and reliability in terms of MTBF. Records of these assessments were captured in the RAM performance assessments to provide a coherent record of the changes applied as the system matured throughout its lifecycle and the rationale for changes and optimisations.
Perhaps one of the novel aspects of optimisation throughout the system lifecycle came from the stage of assessing system value. This was done via the verification and validation of achieved system performance against the aforementioned RAM requirements. Parallel to the testing and commissioning phases of the project, the MMD and Crossrail RAM teams initialised the DRACAS process wherein DRACAS relevant events were captured, assessed, and effects were fed back into the RAM performance assessments. This provided added value by replacing theoretical information with empirical information from the operational systems which provided assurance that the systems were compatible and ready for use at testing, commissioning, deployment, and operation stages.
3.2 Indirect Outcomes
The Indirect Outcomes of the successful application of RAM engineering to the Crossrail project are a reflection of the positive impact that has resulted from the delivering of a new, state-of-the-art railway. The addition of this railway to London’s transportation infrastructure and networks has resulted in a number of outcomes directly related to the operations, efficiency, resilience, connectivity, and performance of the existing transport network. This is direct result of the optimisation of system RAM performance throughout the project lifecycle.
It is projected that this new East-West rail link across London will increase central London’s rail capacity by 10%[6]. A key consequence of this increased capacity is the improved reliability and resilience of the overall public transport network since the addition of a new line provides additional levels of redundancy in the event of failures or disruption on other parts of the system. This has been enabled by the initial definition and quantification of the RAM performance requirements, alongside the increased confidence in the system performance provided by the verification and validation of the aforementioned RAM requirements being met.
It has been estimated that the Elizabeth line will bring an additional 1.5 million people within a 45 minute commute of the existing major employment centres of the West End, the City and Canary Wharf[7]. Additionally, the railway will provide increased access and connectivity between key transportation centres and interchanges, such as Heathrow Airport, Paddington Station, Liverpool St Station, Stratford Station and the future Old Oak Common station (for direct connection to the under-construction High Speed 2). The incorporation of Crossrail into the already complex London rail transport network is a clear example of the potential of Systems Thinking in addressing not only the integration challenges of the technical rail systems within the project, but more importantly of the intricacies of the social and natural environment upon which Crossrail depends.
This improved connectivity is expected to have a positive impact on the use of public transport by people with restricted mobility. All Elizabeth line stations have step-free access from street level to platforms, with stations from Abbey Wood to Paddington being step-free from street to train. The majority of interchanges between Elizabeth line and other LU and national rail services are step-free to accommodate people with restricted mobility. Crossrail have estimated that a total of 95% of journeys will have step-free origin and destination stations. The holistic approach provided by Systems Thinking transforms the delivery of such a complex transport network. Meaningfully connecting the technological system to the physical, psychological and emotional needs of the end user who will use it is an essential part of developing new solutions, improving the system’s function.
While it is possible to quantify these outcomes, it is important to highlight that the extent of the impacts that the Elizabeth Line will have on its environment extends beyond the Indirect Outcomes outlined in this section.
3.3 Induced Outcomes
The Induced Outcomes reflect how RAM engineering involvement has contributed to development of Social, Economic, and Environmental outcomes external to the immediate scope of the project. These are the holistic, less tangible purposes for a project that are induced via the previous Direct and Indirect Outcomes. These are outcomes such as aligning with the government’s overall strategy toward sustainable development; The Mayor of London’s strategies for transport, Capital infrastructure development, and sustainability; The Crossrail Sustainability Strategy[8]; and even the overarching United Nations Sustainable Development Goals (SDGs) that the project contributes to.
The assessment of the system value against the highest level of sustainability requirements, which can be seen to be aligned to the tail end of the Systems Engineering V Lifecycle diagram. Utilising the Brundtland[9] definition of sustainability and sustainable development, “Development that meets the needs of the present without compromising the ability of future generations to meet their own needs”, it is necessary to subdivide this definition to allow more specific analysis. In wider sustainability literature there are a number of ways of categorising sustainability, with an example of some leading sustainability categorisations provided in Figure 7.
Figure 5 shows that increased rail capacity and improved connectivity between key transport hubs can be seen to result in induced impacts across all four identified Induced Outcomes, whereas increased accessibility and reduction of congestion appear slightly more targeted.
Perhaps the most obvious Induced Outcome, and one which is presented as being impacted by all Indirect Outcomes, is that of SDG 9 – Build resilient infrastructure, promote inclusive and sustainable industrialisation and foster innovation. Crossrail is by definition a prime example of resilient transport infrastructure being developed with the power of members of Industry, both Small and Medium Enterprises (SME) and large multinationals, and the innovative approach to RAM engineering discussed in the previous section.
This links well into SDG 11 – Make cities and human settlements inclusive, safe, resilient and sustainable. The Indirect Outcomes feeding into this SDG result in making high quality public transport more available to not only a huge portion of the population in Southeast England, but also people with restricted mobility who have previously faced barriers to inclusion. However, congestion is a problem for more than just the people with restricted mobility. The reduced congestion across the transport network will also benefit the mental health and wellbeing of all inhabitants of London and benefit other vulnerable groups of society who are statistically more likely to be dependent on the public transport systems in London.
Figure 7. Selected Leading Sustainability Categorisations
Furthermore, and tying in both the TfL Sustainability Strategy principles of Economic Progress and SDG 8 – Promote sustained, inclusive and sustainable economic growth, full and productive employment and decent work for all, increased rail capacity and Improved connectivity affects the greater public’s perception of the available transport options. This is believed to induce behavioural changes moving away from more carbon-intensive modes of transport and toward increasing utilisation of more sustainable public modes of transport. Particularly at a time post COVID-19 when tourism and city centres are experiencing unprecedented challenges, these behavioural changes will present opportunities for new facets of sustainable economic growth to emerge in communities that may otherwise have been negatively impacted.
4 Lessons Learned and Recommendations
4.1 Lessons Learned
Based on the discussion and analysis of results in the preceding section, the following lessons have been identified:
- Integrated Systems Thinking, such as that embodied within this RAM workstream provides benefits not only for the delivery of project outputs (i.e. delivery of components, parts, and systems), but also for the delivery of the overarching desired Direct, Indirect and Induced Outcomes of a project.
- While a practitioner of Systems Thinking may be more accustomed to holistic approaches to projects, different stakeholders may be less familiar with this. As a result of this, there may be clashes of perspective about what amounts to be a priority at any given time. For this reason the RAM workstream had the opportunity to progress through influencing and collaborating with others.
- Just as systems within Crossrail have complex interfaces, interdependencies, and emergent properties, so do the effects of the outcomes of the project as a whole.
- The use of empirical data during the testing and commissioning phases of a large interdisciplinary project, to verify and validate the RAM performance modelling predictions, is a benefit not only in increasing the confidence in the systems performance, and in the analytical tools and methods used to derive the theoretical performance estimates.
When considering the outcomes of the RAM activities carried out as part of the Crossrail project, it is evident that RAM provided a significant contribution to the implementation of the Systems Thinking approach that was adopted for the delivery of such a railway. While the lessons learned highlight some of the areas which have room for improvement in the context of RAM engineering for complex multidisciplinary projects, it can be said that any improvements in the field of RAM will, as a direct result, have a positive impact on the wider application of Systems Thinking as a whole.
4.2 Recommendations
Based on these Lessons Learned, the following recommendations have been made:
- Executive Sponsorship, in this instance by the TfL and ATC leadership teams, is key in order to create an environment that facilitates collaboration and gives credence to the holistic Systems Thinking activities. Without this key input, there is the risk of deliverables being deferred until they are on the critical path.
- It is essential to understand the Problem Space and the Desired Outcomes. In terms of utilising Systems Thinking collaboratively with those less familiar with the theory, it may prove to be beneficial to really focus on the common-sense aspect of the “Big Picture thinking and application of common sense to projects” [5] concept of Systems Thinking. By articulating the processes into a less technical common sense language that constantly links back to the Desired Outcomes, it may prove more effective in gaining more continuous buy-in from colleagues with fewer ebbs and flows.
- It may be beneficial to attempt to utilise those working on Systems Thinking aspects of the project to teach and empower others on how to implement Systems Thinking themselves rather than taking complete ownership and control of it all. This could prove beneficial particularly when large amounts of deliverables are expected at similar times around certain deadlines.
- System of Systems interdependencies and resulting emergent properties is a developing area of study. Early adoption of this may prove beneficial and significantly bolster resilience of future projects in constantly evolving highly complex environments.
- The application of Systems Thinking and RAM performance analysis throughout the project lifecycle allows for an increased level of confidence in the design and architecture of the project systems. Since the quality and accuracy of the modelled predictions increases with the level of maturity of design, a comprehensive understanding of system performance has been developed before the time-critical stages of testing and commissioning are reached.
5 Conclusion
This paper has been developed to explain how RAM performance engineering has promoted commitment to the social outcomes of this project, to the benefit of clients, end users, and society as a whole.
Using a Theory of Change Framework has provided insight into how the output of the RAM workstream has not only contributed to the delivery of a complex multidisciplinary project, but also the resulting outcomes. These outcomes were assessed on three distinct levels: Direct, Indirect, and Induced Outcomes. Interdependencies between the RAM workstream and the Outcomes, though highly complex, have been explored to a fitting level of granularity.
As a result of the discussion and analysis, this paper has presented a number of lessons learned and potential recommendation to implement them, primarily revolving on the adoption of comprehensive Systems Thinking, such as RAM engineering. The application of RAM engineering on Crossrail has proven to provide benefits not only to project delivery, but also to the realisation of desired system outcomes. Embedded Systems Thinking throughout the project lifecycle presents an opportunity to control and capitalise on emergent properties that arise in an increasingly interconnected world.
References
[1] BSI. BS EN 50126-1, “Railway Applications. The Specification and Demonstration of Reliability, Availability, Maintainability and Safety (RAMS), Generic RAMS Process”. BSI Standards Limited; 1999.
[2] The Safety and Reliability Society (SaRS). “Applied R&M Manual for Defence Systems: Part D – Supporting Theory: Chapter 8 – Reliability Growth Models”, GR-77 Issue (Version 2.5), 2012: 2-9.
[3] Crossrail Limited. CRL1-XRL-O8-GUI-CR001-50013, “Integration – O&M Interface: DRACAS User Guide”. 2016: 2-14.
[4] P. Brest. “The Power of Theories of Change”. Stanford Social Innovation Review. Stanford: Stanford Social Innovation Review; 2010: 47-51.
[5] INCOSE UK. “Z1 Guide: What is Systems Engineering? Creating Successful Systems”. London: UK Chapter of the International Council on Systems Engineering; 2020.
[6] Steer Davies & Gleave Ltd. “Crossrail: Summary Transport Case Report”. London: Cross London Rail Links Ltd.; 2005: 45-45.
[7] Crossrail Limited. “Crossrail Business Case: Summary Report”. Transport for London (TfL); 2010: 8.
[8] Crossrail Limited. “Crossrail Sustainability Strategy”. London: Transport for London (TfL); 2013.
[9] World Commission on Environment and Development. “Our Common Future”. Oxford University Press; 1987.
-
Authors
Paul Muchatuta BEng MSC MIET - Mott MacDonald
RAMS Engineer, Crossrail C610 Systemwide Main Works, Assistant Systems Engineer, Mott MacDonald
Tomas Cuesta Brooke-Turner MEng MIET - Mott MacDonald
RAMS Engineer, Crossrail C610 Systemwide Main Works, Assistant Systems Engineer Mott MacDonald Ltd.