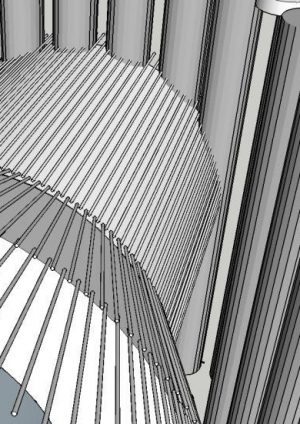
Stabilisation of soil pillar adjacent to Eastern Ticket Hall at Bond Street Crossrail Station
Document
type: Technical Paper
Author:
Hilary Skinner MA (Cantab) FICE, Vicky Potts MA MSc PhD, Adrian St.John, BEng (Hons) Eur Ing FICE CEng, Damian McGirr BEng (Hons) MICE CEng, ICE Publishing
Publication
Date: 03/11/2014
-
Abstract
Sprayed concrete lining (SCL) tunnelling alongside the secant pile walls forming the Eastern Ticket Hall (ETH) box at Bond Street station left a narrow pillar of clay, less than 1m wide in places, between the piles and the tunnel lining. Since the tunnelling was to occur after excavation of the box, the stability of this soil pillar during excavation was identified as a concern. Analysis undertaken as part of a due diligence review of the lining design indicated that there was indeed a high risk of overstressing in this area with potential for a collapse mechanism to develop during SCL excavation.
This paper describes this identification and modelling of the ground stability risk, and also the selection and design process that was employed in the development of a detailed temporary works scheme to mitigate that risk. The difficulties associated with the implementation of that design in a highly constrained location are also discussed. A significant degree of collaboration between the construction and design teams enabled amendments to be made to alleviate some construction difficulties without compromising the effectiveness of the soil stabilisation scheme.
Ultimately it was decided to use a grouted pipe arch at the breakout from the box and, where a pipe arch was no longer feasible due to the tunnel geometry, an innovative solution to create a mattress of reinforced soil above the tunnel was developed using overlapping arrays of grouted spiles installed from the shaft.
The works undertaken to stabilise the soil pillar adjacent to the ETH at Bond Street exemplify the successful use of an integrated approach to design and construction to mitigate potential ground instability during SCL excavation.
-
Read the full document
List of Notations
BFK Bam Ferrovial Kier JV (Contractor)
DAL Donaldson Associates Ltd (Contractor’s Temporary Works Designer)
DDR Due Diligence Review
ETH Eastern Ticket Hall
SCL Sprayed Concrete LiningIntroduction
Crossrail is Europe’s largest construction project, and will transform rail transport in London, increasing capacity by 10%, supporting regeneration and cutting journey times across the city. The Crossrail route will run over 100km from Maidenhead and Heathrow in the west, through new tunnels under central London to Shenfield and Abbey Wood in the east. There will be 38 Crossrail stations including 9 new stations at Paddington, Bond Street, Tottenham Court Road, Farringdon, Liverpool Street, Whitechapel, Canary Wharf, Custom House and Woolwich.
A joint venture of BAM Ferrovial Kier (BFK) was awarded the Crossrail contract C300/C410 “Western Running Tunnels and Station Caverns” in January 2011. This comprised the construction of two 6.2km tunnel drives between Royal Oak and Farringdon and the construction of sprayed concrete lining (SCL) tunnels for Bond Street and Tottenham Court Road Station tunnels. Donaldson Associates (DAL) was appointed as BFK’s SCL designers for these works. These two parties had a clear responsibility for excavation stability. DAL’s scope of work comprised a Due Diligence Review (DDR) on the primary lining design, which formed part of the permanent works, and the design of “Toolbox items” and any other necessary measures required wherever an unacceptable risk of instability during excavation was identified. Further to this, a 24/7 team of Senior SCL Engineers and SCL Shift Superintendents from Donaldson Associates was embedded into the BFK Engineering team.
This paper relates to a specific element of these works at Bond Street Station – the construction of LCE1 and LCE2 tunnels. A plan of the affected area is shown in Figure 1.
Figure 1: Layout of Eastern Ticket Hall at Bond Street Station
The Eastern Ticket Hall (ETH) was formed using a secant piled wall by another Crossrail contract, with pile depths of up to 45m below ground level. Female piles terminated just above the tunnel horizon, meaning that at tunnel level the piles were contiguous. Temporary internal piled walls divide the ETH into three parts; the north-west shaft, the masterplan shaft, and the rest of the ETH, see Figure 1. This was done to allow early access for SCL works from some parts of the box. As part of the advance works (prior to TBM arrival at Bond Street) SCL tunnelling was to commence working westwards from the north-west shaft. Tunnels LCE1 and LCE2 were to be constructed parallel to the ETH wall, mostly alongside the recently-constructed masterplan shaft. At some locations, the extrados of the primary lining for LCE1 and LCE2 was less than 1m from the piles forming the ETH wall.
It was realised at an early stage in the DDR that this would leave a narrow pillar of clay between the tunnel and the piles, and that this would potentially be vulnerable to overstressing during SCL tunnel excavation. The installation of the piles in the ETH and subsequent excavation of the north-west and masterplan shafts may have caused disturbance of the ground and lowered the in-situ stresses, both of which increased the risk of overstressing during excavation. This concern was compounded by LCE2 being the largest tunnel profile to be excavated at Bond Street, with an excavated horizontal span of over 12.8m, see Figure 2. The risks associated with construction of these tunnels and possible solutions were discussed at a series of workshops between MM, BFK, Crossrail and DAL.
Figure 2: LCE2 cross section
This paper describes the assessment of the risks at this location, and describes the development of a safe, practical and effective scheme by DAL and BFK to mitigate these risks.
Assessment of Risk
Having identified a potential concern over stability at this location, a 2D finite element model was developed using Zsoil (2011) to investigate the stress conditions in the soil pillar between the tunnel and the piles at various construction stages. Two sections, across LCE1 and LCE2, were chosen for the analysis as shown in Figure 1. The analysis and the results are described in the following sections.
Ground Conditions
The stratigraphy at this location is indicated in Figure 3, along with the pore water pressure profile in the London Clay, which is underdrained by the lower chalk aquifer.
Figure 3: Modelled ground conditions for LCE1 and LCE2 (including location of LCE2)
A coupled consolidation analysis was undertaken using a hardening soil model for the clay materials at or near the tunnel horizon to replicate reasonable small strain stiffnesses during excavation. For the superficial soils, a Mohr-Coulomb constitutive model was considered adequate. The parameters were chosen to give a reasonable prediction of the behaviour, and were not factored. The geotechnical parameters used in the finite element analysis are summarised in Table 1. Permeability was selected to replicate the known insitu water pressure distribution.
Table 1: Geotechnical parameters used in FE analysis
Additional analyses were undertaken varying the strength and stiffness parameters of the soil to assess the sensitivity of the lining response to changes in ground conditions.
Sequence of Construction
The FE model replicated the following sequence of construction:
- Generation of initial stresses and water pressure distribution (based on K0 and Kw values in Table 1)
- Installation of the ETH piles – these were wished-in-place
- Excavation of the north-west shaft and masterplan shaft. A sequence of successive excavation to 0.5m beyond propping levels shown on the design drawings, followed by installation of the prop, was adopted for the excavation of all 5 levels within the shaft down to the base slab level
- Casting of the base slab
- Excavation and spraying of LCE1/2, according to the design sequence (top heading and invert for LCE1, pilot, top heading, bench and invert for LCE2)
Modelling of SCL Tunnel Construction
In order to model tunnel excavation steps such that the 2D model was representative of 3D tunnel excavation, ground stress redistribution was modelled by allowing 30% of the internal stress within the elements to be excavated for the pilot and top headings, to be carried outside the model and 70% of the stress redistributed to the model itself. For the bench and invert these figures were adjusted to 20% and 80% respectively to take account of the prior excavation steps. The time over which the redistribution was allowed to occur coincided with approximately 10m of tunnel construction. The sprayed lining gained stiffness and strength according to the modified J2 curve.
Results of FE Modelling
Figures 4 and 5 show the stress level contours at the end of tunnel excavation for LCE1 and LCE2 respectively
Figure 4: Stress level contours at the end of excavation of LCE1
Figure 5: Stress level contours at the end of excavation of LCE2
The results suggested that in both cases more than 85-90% of the available soil strength in the London Clay soil pillar between the tunnel and the wall would be mobilised at the end of construction. This was highest for LCE2, where the results suggested that 96% of the available shear strength was utilised (Figure 5). At 100% utilisation, the soil would begin to yield and in a situation such as this significant deformations would be likely to develop. The extent of the highly stressed soil in Figure 5 was also of concern.
These results indicated that – in an unfactored analysis – the soil pillar was highly stressed and approaching full mobilisation of the soil mass. This was a result of the relaxation of horizontal stress locally following the shaft excavation and then the tunnelling work. The modelling assumed that the London Clay was homogenous throughout its mass, and did not take account of any fissuring. The possible presence of adversely-oriented fissures presented an unacceptable risk of instability of the soil column during excavation.
Mitigation of Risk
Having identified a potential risk to the stability of the excavation a solution was required to mitigate this risk. Any solution needed to take account both of physical constraints, and due to the proximity to adjacent works there was potential for numerous interfaces with other designers and contractors. These are discussed in detail in this section.
For the SCL tunnels constructed under contract C300/C410, the primary lining was designed to form part of the permanent works as a composite SCL lining and therefore any solution could not compromise the integrity or performance of the SCL primary lining. The complex geometry of this part of the SCL tunnelling further limited the options for installing support measures.
Installation of pre-support measures from within the ETH was also complicated due to the box being only partially excavated at the time of construction of LCE1 and LCE2. The north-west shaft would clearly need to have been excavated to allow tunnelling for LCE1 and LCE2 to commence; in contrast, the masterplan shaft (being constructed by an adjoining Crossrail contractor) was programmed to be excavated concurrently with the construction of LCE1. The remainder of the ETH would be excavated at a later stage. Furthermore, it was not possible to impose any load on the ETH piles as these had not been designed to allow for this. Any solution therefore needed to be independent of the piled wall structure.
The constraints for the three parts of the tunnel (LCE1, flared transition from LCE1 to LCE2 and LCE2) are summarised in Table 2.
Part of tunnel Constraints LCE1 Accessible from the already-excavated north west shaft. Transition Flare commenced at approximately 8m from the north-west shaft. Support measures to be installed from within tunnel or masterplan shafts (NB. ETH not excavated) LCE2 Support measures to be installed from within tunnel (or masterplan shaft, if constructed). Table 2: Constraints on installation of support measures
In practice the support measures were selected to both address the risk of soil failure and to address the constraints for installation. Support measures were preferred that could be installed ahead of tunnel excavation, as delay in achieving ring closure was seen as increasing the risk to excavation stability and potentially increasing settlement.
For LCE1, installation of a grouted pipe arch from the north-west shaft would provide protection against both local and global potential instability (risk of falling blocks and failure of the soil pillar respectively). This could be safely installed from the north-west shaft, and a portal arch was designed to sit inside the piles of the north-west shaft to support the pipes independently of the piles.
Provision of support measures for the transition and LCE2 was particularly difficult. The complex geometry of the transition (see Figure 6) was not well suited to installing pipes from the ETH or spiles from within the tunnel. Besides which, spiling would only provide protection against potential instability local to the tunnels; it would not be able to support the vulnerable soil pillar.
Significant amendments to the permanent works design were ruled out due to spaceproofing requirements and programme constraints. Soil stabilisation from the surface was practically impossible due to logistical constraints and the presence of a temporary shaft with Tube à Manchette (TaM) arrays for the compensation grouting. Reducing the length of the excavation rounds was discounted as it was considered that these would make excavation and spraying more difficult without accelerating ring closure and therefore any benefit would be limited.
Consideration was given to using tensioned cables between the pilot tunnel and the piled wall to raise the horizontal stresses in the soil pillar and improve its strength. However this inevitably involved an interface with both the primary lining and the piles would need assessment and agreement from the designers of both of these elements. The spacing involved in installing the cables between piles did not suit the round lengths proposed for the SCL tunnelling. To prevent the primary lining from being adversely affected would have meant releasing the tension in the cables at the critical moment of excavation and this solution was therefore not considered appropriate.
The preferred solution was to create a reinforced earth mattress above the tunnel to intercept the vulnerable soil column and enhance the capacity of the soil to spread loading over a wider area. This was to be achieved using an array of overlapping grouted pipes installed near-horizontally from the masterplan shaft. This approach had numerous advantages:
• The complex geometry of LCE2 and the transition did not interfere with the installation
• As a pre-support system the grouted spiles could be installed offline
• No delay to achieving ring closure
• No impact on adjacent permanent works
• Additional “Toolbox items” (e.g. spiles, pocket excavation) were still feasible if problematic ground conditions were encountered locallyDesign Development
The design of the pipe arch followed conventional methods, with loads determined from the FE model and based on an agreed excavation sequence. The design was able to allow for the installation of grouted spiles above the transition, if needed for local ground stability (e.g. if ground found to be highly fissured). This is shown indicatively in Figure 6. As the pipes were to be installed in close proximity to the excavation, the key issue was to ensure the pipes would not protrude into the transition.
Figure 6: Indicative sketch of grouted pipe arch above LCE1 allowing for installation of spiles above transition if needed
The reinforced soil mattress was designed in two ways. Firstly, the degree of improvement in soil parameters and the depth over which this would be required was investigated using the FE model described in Section 3. A modest improvement in soil parameters (around 10%) was required over a 1m band above the tunnel to disrupt the formation of the potential failure mechanism. The potential for local failure would remain but could be addressed by the pre-designed toolbox measures. A mattress was judged to be created provided that a system of reinforcing elements could be inserted that crossed each other more than once over the required depth, as indicated in Figure 7. A layout for grouted pipes was devised, which would not be required to carry structural load but to enable the soil to act as a more coherent mass. The pipes would be installed from the masterplan shaft, from between the contiguous piles, sufficiently above the transition and LCE2 that spiles could be installed if necessary. The pipe size was selected such that it was large enough to enable drilling of around 12m, but sufficiently small to facilitate drilling at angles in between the existing piles with a free space of around 150mm. Self-drilling pipes of 76mm diameter were judged to fulfil both requirements.
Figure 7: Indicative sketch showing overlapping array of grouted spiles above LCE2
In order to install these safely and to avoid the masterplan prop slabs and/or the requirement for additional temporary working platforms, it was proposed that the pipes were installed at an angle to the horizontal. The lengths and angle of the pipes was intended to avoid the TAMs already in place 4m above the tunnels.
Figure 8 gives an indicative elevation showing the relative location of the temporary props in the masterplan shaft, the drilling rig, the position of the TaM’s and how these factors combined to constrain the installation of the grouted spile array, along with the proposed solution. Figure 9 shows the design layout of the grouted spile array above LCE2.
Figure 8: Elevation showing installation constraints for grouted spile array
Figure 9: Design Layout of Grouted Spile Array above LCE2
Residual Risk
The construction team had been consulted extensively during the design development period, yet there remained concerns that, on such a large project with multiple shifts, the design philosophy and residual design risks associated with a novel scheme such as this may not have been fully appreciated by the wider construction and supervisory teams. Designer-led toolbox talks were considered to be the most effective means of communicating these issues, and this innovation was sufficiently successful to be rolled out elsewhere on the project.
Construction
In spite of the best efforts of all parties during the design process, some difficulties were still encountered during construction of the grouted spile array above LCE2. In particular, during installation of the first high level grouted spiles, grout ingress was observed from one of the nearby TaMs. A further review was undertaken to assess the as-built TaM positions, the design locations of the spiles and the drilling tolerances to assess their proximity and the likelihood of clashes. As a precaution the separation between the two was increased to reduce the likelihood of recurrence. The length reduction of some pipes was anticipated to reduce the effectiveness of the mattress, but not in the key area between tunnel crown and masterplan shaft. The full time site presence of Donaldson staff within the BFK Engineering team enabled rapid and effective communication of issues and decision-making without delaying site progress.Once drilling from the masterplan shaft had commenced it also became evident that the limitations on the location of the drilling rig during operation were more onerous than anticipated, due to the working room requirements for the drilling rig being used and the awkward nature of the space in the masterplan shaft. Not all of the specified pattern of spiles could be installed and therefore a rapid re-assessment was needed. This was the case in the north-west corner of the masterplan shaft, where working room was particularly limited, and meant that spile coverage above the final few metres of LCE2 would be particularly difficult to achieve. Figure 10 illustrates the awkward and congested nature of the site at the time these works were being undertaken.
Figure 10: Site Layout at the time of installation of the grouted spile array
The geotechnical records obtained from the enlargement of LCE1 and the start of LCE2 (above which it was feasible to install the grouted spile array) indicated that the ground conditions were likely to be better than allowed for in the design. This was confirmed by further site visits to observe the geotechnical conditions during enlargement of early parts of LCE2 and logging of the excavation of the pilot tunnel through the affected areas. In view of this, the design was reviewed to confirm the requirements if the ground conditions remained favourable. It was found that the coverage of the array could be reduced where the distance between the masterplan shaft and LCE2 increased provided that the ground conditions remained favourable.
As more information became available following excavation of the pilot tunnel through LCE1 and LCE2, and as excavation of LCE2 progressed under the reinforced soil mattress, confidence in the ground conditions grew and the design was adapted accordingly. The final plan layout of the grouted spile array once the various constraints had been taken into account is shown in Figure 10.
Conclusion
Both tunnels LCE1 and LCE2 were constructed successfully with no block failures or excessive movement either within the tunnel or of the surrounding assets. Whilst the pipe arch solution over LCE1 was a tried and tested method, the grouted pipe ‘mattress’ over the transition and LCE2 was a novel approach and driven out of necessity as a result of the challenging geometry and significant physical and logistical constraints at this location.
The combination of a pipe arch and an innovative grouted spile array as a pre-support system at this complex location had numerous benefits to the project, but the principal advantages were that there was no adverse impact to any of the adjacent permanent works, and that no further in-tunnel measures were required during excavation, leading to more rapid ring closure. Furthermore these works could be carried out offline, without having any impact on the SCL tunnelling.
Whilst the design of measures could be carried out readily, the installation constraints drove the choice of solutions, and the installation issues required close consultation between installer and designer together with careful re-evaluation of the design. The benefit of embedding key members of the design team onto site and carrying out careful observation and recording allowed rapid re-analysis of the design based on site observations and a reduction in the requirement for installation to the benefit of the project. This highly effective communication between the design and construction teams resulted in the successful and timely re-evaluation of the design wherever this was required by the challenging conditions encountered on site.
-
Authors
Hilary Skinner MA (Cantab) FICE - Donaldson Associates Ltd
Donaldson Associates Ltd
Vicky Potts MA MSc PhD - Donaldson Associates Ltd
Donaldson Associates Ltd
Adrian St.John, BEng (Hons) Eur Ing FICE CEng - BAM Ferrovial Kier
Adrian St.John is a Fellow of the Institution of Civil Engineers and a Supervising Civil Engineer. He has spent most of his 24 year career working on major infrastructure and tunnelling projects in the UK and overseas, including the Brighton Stormwater tunnel and High Speed 1 at St Pancras. He spent 6 years as Chief Engineer for BFK Joint Venture who were the main contractor on Crossrail contracts C300, C410 and C435, and is the Design Director for Carillion Eiffage Kier (CEK) Joint Venture bidding for the forthcoming HS2 main civil works.
Damian McGirr BEng (Hons) MICE CEng - Donaldson Associates Ltd
Donaldson Associates Ltd